- solutions
- industries
- about us
- resources

Resources - Blog - Business
May 21st, 2024
Sustainability
Leading the Charge: Solar Photovoltaics in the Energy Transition
Costanza Tinari
As we advance our ability to gather and analyze data on the environmental impacts of energy production, the urgency to decrease our reliance on fossil fuel is as clear as ever. In fact, over the past two decades the deployment of renewable energy infrastructure has doubled. Among these renewable sources, solar power stands out as a key player. Currently making up 5.4% of global electricity generation, solar photovoltaics (PVs) are positioned for exponential growth, projected to increase sixfold in the next decade (IEA, 2024).

A Brief History of Solar Photovoltaics (PVs)
Humans have been harnessing the power of the sun ever since the 7th century BCE, using large windows to capture sunlight to heat their homes, and using lenses to light fires. In the 1700s researchers had investigated how to harness solar energy more effectively. These efforts lead to the invention of the first solar oven and solar powered steam boats. The breakthrough discovery occurred in 1839, when a 19-year old french physicist, Edmund Becquerel, studied the photovoltaic (PV) effect and noticed that exposure to light resulted in a cell to produce electricity. Sixty-four years later, the photoconductive property of selenium was discovered, leading to the development of the first PV cell in 1883 - but with an efficiency of only 1%.
The Evolution and Growth of Solar PV Technology
Today, thousands of patents for solar harnessing devices exist, with efficiencies up to 40%. Solar technologies are divided into two main categories: concentrated solar power (CSP) and PVs. The former utilizes mirrors or lenses to concentrate sunlight onto a small area, while the latter converts sunlight into electricity using semiconductor materials. This blog will focus on PVs, exploring how they are made, how they work, and their role in the energy transition.
Components of a Solar PV Module
PVs are made up of modules (aka panels), respectively made up of several components including solar cells, glass panels, the mounting structure, and cabling, as represented in Figure 2, below. On average, the efficiency of PVs lies between 20 and 30%, meaning that they successfully convert that portion of solar radiation into power, and have an average lifespan of 25-30 years. Solar radiation resulting from the sun’s fission reactions reaches the earth in varying amounts depending on atmospheric conditions, latitude, and season. In the UK, for example, the average annual irradiance is 100 W/m2.
A 1m2 PV in the UK would therefore convert ~25% of that radiation, producing 25W over a year. In its lifetime, it would produce ~625W, powering around 20 fridges!

Types of Solar PVs: Crystalline Silicon and Thin-Film Technologies
There are different types of PVs, their classification depends on the minerals that make up their cells. The most popular types are the ‘first generation’ PVs that use crystalline silicon (c-Si) based cells (accounting for >98% of production in 2022). These can be either monocrystalline or multicrystalline, so either made up of single silicon crystals, or of multiple smaller crystals (which improves cost efficiency but decreases performance). The ‘second generation’ PVs are referred to as ‘thin-films’ and are made by depositing a layer of semiconducting material onto a substrate like glass, plastic or stainless steel. There are three main types of thin-film PV, classified based on the PV semiconducting material used: 1) Cadmium-Tellurium (CdTe), 2) Amorphous Silicon (a-Si), 3) Copper-Indium-(Gallium)-Selenium (CI(G)S), of which CdTe is the most prevalent by a significant margin.
How Solar PV Cells Generate Electricity
Let's take c-Si PV. The three minerals needed to make a panel are silicon, boron, and phosphorus, essential to produce an electrical field! The Si is mixed (‘doped’) with boron to create a positive charge (P+), and with phosphorus to create a negative charge (N-). And this is where the sun comes into play: when sunlight, consisting of photons, strikes the solar cell, it transfers energy to the semiconductor material, which causes some of the electrons in the semiconductor's atoms to become 'excited,' gaining enough energy to break free from their bonds with the atoms becoming 'free electrons', able to move freely within the material. However, the diffusion of electrons from one side to the other creates a region where there are no charge carriers, known as the ‘depletion zone barrier’. By connecting the cell to an external circuit the flow is re-established, producing an electrical current. As it moves through the conductor, this current produces electricity!
Sourcing Essential Minerals for Solar PV Production
Silicon comes from silica sand that is mined from quartz-rich sand or rock. The raw element, second-most abundant found in the earth’s crust, is extracted from the ore by being heated in a furnace with a source of carbon. Then, it’s turned into a fine powder, purified, and processed further to finally produce semiconductor-grade Si! Boron and phosphorus are produced from borate and phosphate rock respectively, then processed.
Thin films, on the other hand, reduce or replace silicon with rare materials with similar semi-conducting properties, like cadmium and tellurium. These two elements are obtained as a by-product of zinc and copper production respectively, then powdered and purified.
Environmental Impact of Solar PV Production
Although it produces renewable energy, this technology is not inherently renewable: the finite minerals that need to be extracted from the ground and processed into the final products. These operations are energy-intensive and can have significant environmental impacts. It is therefore crucial to leverage our knowledge and expertise to evaluate these impacts, and work towards minimizing them. Proper management of the end-of-life of these materials is also essential, to promote a circular economy within the industry and reduce reliance on raw minerals exploitation. This proactive approach will ensure that the energy transition is genuinely sustainable.
Promoting Sustainability: Life Cycle Assessments (LCAs) in Solar PVs
Life cycle assessments (LCAs) serve as a vital tool in comprehensively evaluating the environmental impacts of solar PV production. By conducting LCAs, we can identify environmental hotspots throughout the entire lifecycle of PV systems, from raw material extraction to end-of-life management. This holistic approach enables us to pinpoint areas of concern and develop effective strategies to mitigate environmental impacts. Furthermore, LCAs provide valuable insights for environmentally informed decision-making, guiding stakeholders in adopting sustainable practices at every stage of a project or product's life. With its ability to highlight environmental trade-offs and promote resource efficiency, LCA emerges as a robust and reliable methodology essential for fostering a genuinely sustainable energy transition.
Costanza Tinari
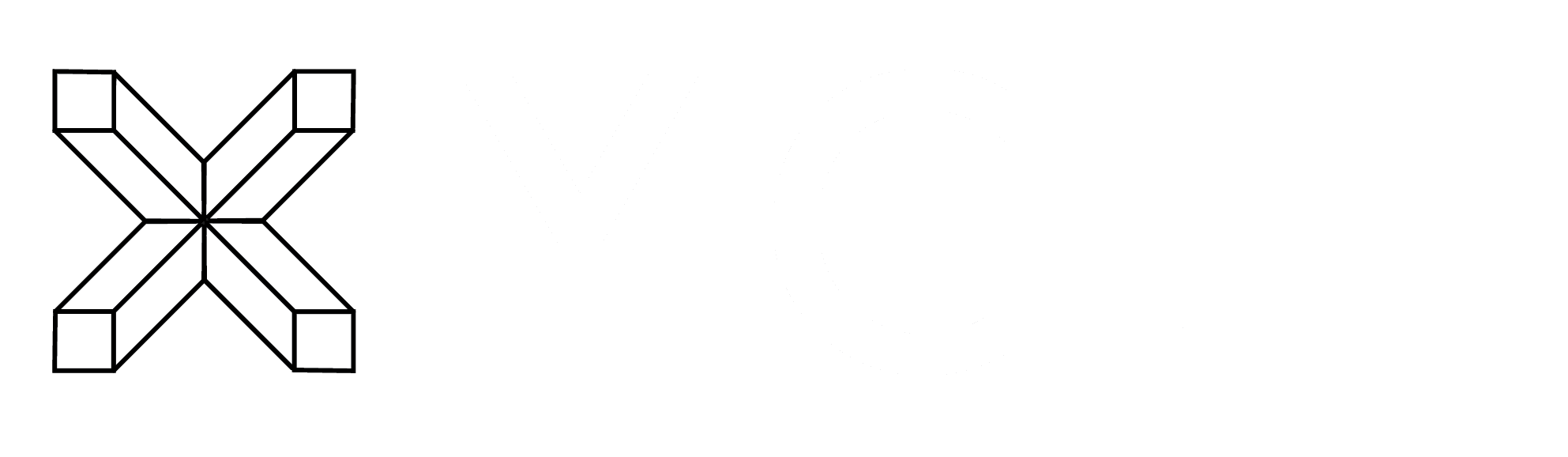